Boston Atomics is a nuclear R&D firm. As experienced advanced reactor designers, we offer insights in system integration, techno-economic analysis, and technology barriers and opportunities for advanced reactors. Our vision is to foster a policy, regulatory, and technical environment that enables advanced reactors to scale and thrive.
We work with our partners to develop cost-reducing technology for new nuclear and provide consulting services to the broader energy industry. In early reactor conceptualization, single technical disciplines often dominate the design philosophy at the cost of other disciplines. This makes realizing performance improvements challenging because changes that improve one dimension often hinder others.
At Boston Atomics, we employ a multi-disciplinary approach, allowing us to more readily identify innovations with realizable benefits. This holistic perspective enables us to see the broader picture of the advanced reactor landscape. Through this blog, we aim to explore and discuss the necessary technological and regulatory developments that will propel the advanced reactor community forward. Our goal is to contribute to the field's evolution from isolated, one-of-a-kind projects to a robust, scalable industry.
Design for constructability
Through reconciling disparate fields: civil and nuclear engineering, we invented a novel reactor concept called MIGHTR. MIGHTR introduces a unique approach to exploiting modular construction techniques for nuclear systems.
It was born from the idea that constructability needs an equal place among other objectives in early reactor conceptualization. Advanced reactors (ARs) and small modular reactors (SMRs), because of their lost economy of scale, need paradigm shifting innovation – ideas that shift the cost curve, not just optimize down the curve.
The MIGHTR concept integrates the largest components of High Temperature Gas Reactors (HTGRs) and rotates them from a vertical orientation to a horizontal one. Under a subcontract through MIT, we embarked on a DOE funded 3 year, $4.9M effort to evaluate the safety systems, main components, core physics, and thermal fluids of our new architecture, which included collaborators from University of Michigan, Argonne National Lab, MPR, and SGH. We continue to incubate MIGHTR in collaboration with universities and national labs, and our website has links to all the publications describing our progress.
Our work developing MIGHTR demonstrates why we believe in designing-for-constructability from the beginning. Civil structures dictate the pace and cost of nuclear construction, so design for constructability is a winning approach, but it typically enters the design process too late, after much of the design architecture is fixed. By this time, you are working down the value engineering curve, not shifting it to unlock real cost reductions.
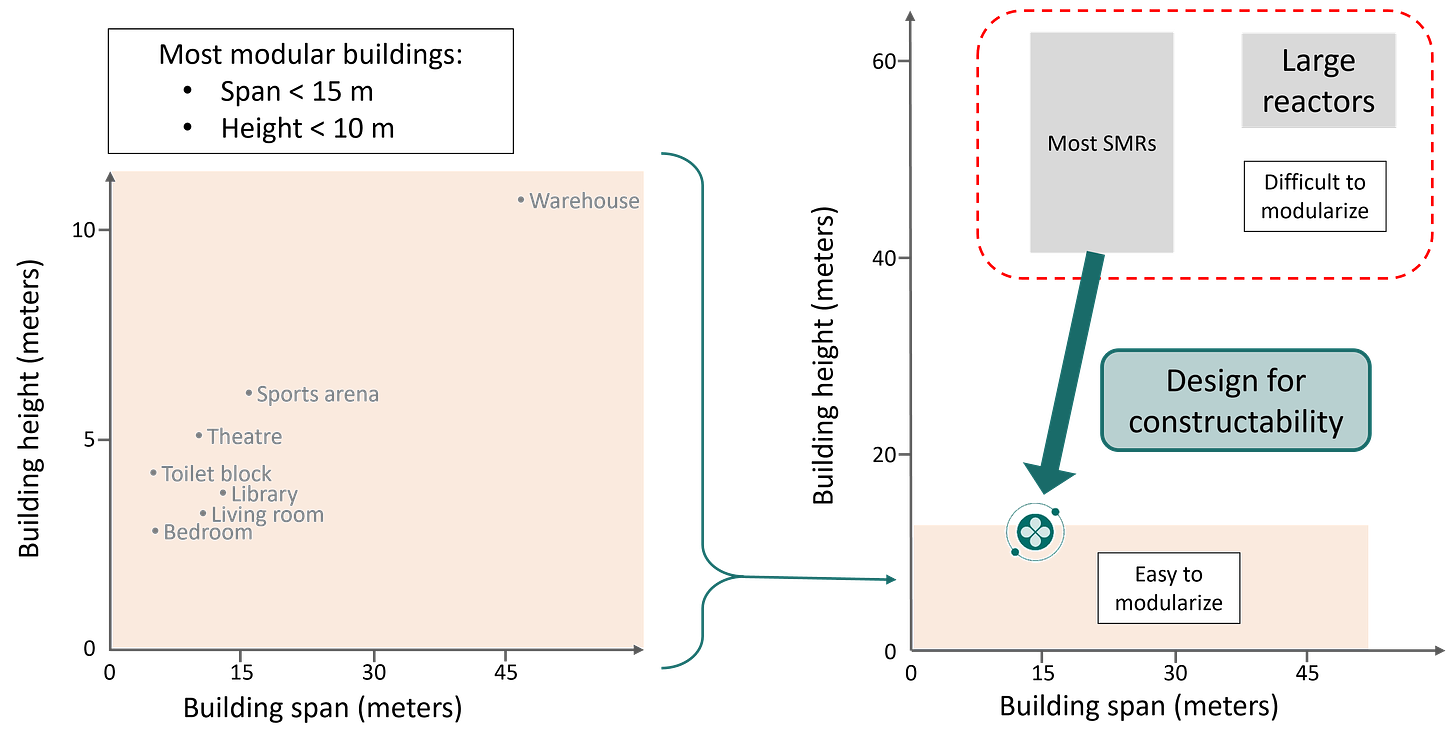
At the height of nuclear construction in the U.S., United Engineers and Constructors published a report on nuclear plant construction costs, and they stated:
The most significant factor dictating construction sequence is gravity. By investigating elimination of the vertical component in plant arrangement, it is possible to substantially change the sequence of erection. The reduction of the vertical component has a further advantageous effect in that it reduces lifting requirements, vertical access for crafts, and related safety and indirect cost considerations.
The potential of our approach is uncertain, but as part of the ARC-20 project we estimated the cost savings to be on the order of 30%.
What is clear, however, is that we are going to hear extensively about the need for value engineering and constructability for ARs and SMRs. That work will be critical, and how well each reactor architecture and firm can value engineer their way down the cost curve will determine their success.
Why an HTGR?
HTGRs are a great complement to water cooled reactors (WCR), because of their relative technology maturity, global development effort, and market opportunity in industrial process heat.
The global HTGR coalition
HTGRs, like many advanced reactors, have many attractive features: passive safety systems, high temperature output and thermal efficiency, reduced emergency evacuation zones, and others. Relative to WCRs, however, they are technologically immature and with nascent supply chains and regulatory readiness. According to the IAEA, today’s global WCR supply chain services >400 reactors, and WCRs are 94% of reactors in construction. Therefore, when selecting an advanced reactor to develop, we wanted to choose one with global development inertia.
The last three decades have seen significant investment in HTGR technology:
The experimental HTR-10 in China (design in 1992, construction in 1995, operation started in 2003)
The commercial demonstration HTR-PM in China (design in 2001, construction in 2012, operation started in 2023)
The Next Generation Nuclear Plant (NGNP) R&D program in the U.S. made progress in regulatory readiness and fuel development.
The GEMINI Initiative and NC2I in Europe focuses on design, fuel supply chain, regulatory readiness, and customer demand.
Commercial ventures: X-Energy, Ultra Safe Nuclear Corp, Radiant Nuclear, and others
The advanced reactor program in France supporting Jimmy
The pebble bed modular reactor program in South Africa
The broad coalition of HTGR development meant that the supply chain, regulation, and technology readiness were outsized relative to other advanced reactor technology, and the odds of commercial success were substantially higher. Further, all the recent deployments of HTGRs have taken place in China, making it in the strategic national interest to develop this capability in the U.S.
In the same vein, we knew that the operational performance of advanced reactors has historically been worse than the water-cooled fleet. Therefore, we wanted to select a coolant with substantial operating history. Water-cooled reactors dominate the global operating experience with 90% of the commercial reactor-years, but gas-cooled reactors have more than 9X the experience that metal-cooled reactors do.
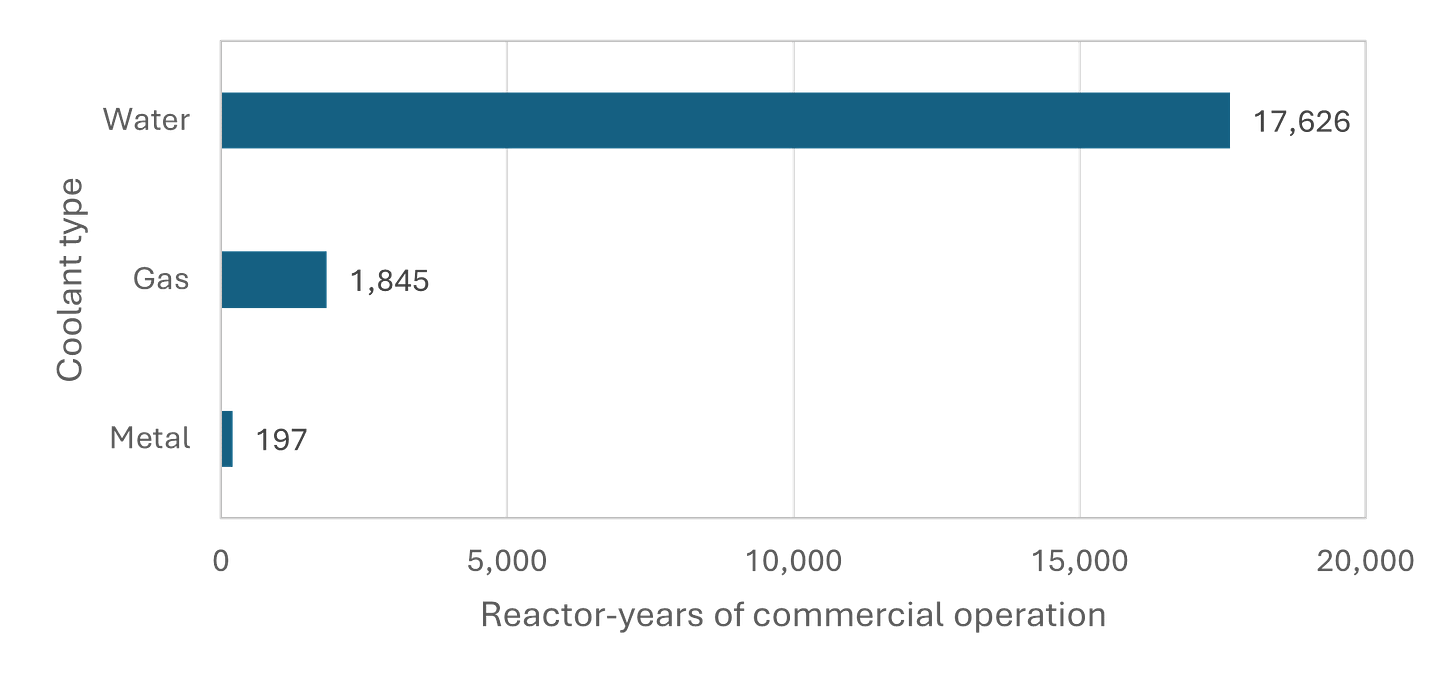
Process heat - valuable market opportunity
In the effort to decarbonize our economy, industrial process heat is an enormous challenge and therefore an opportunity. In a report on Low Carbon Heat Solutions, the Columbia Center on Global Energy Policy found that nuclear could offer the lowest cost source of zero-emission heat for certain temperature ranges. Nuclear power produces heat that is converted to electricity at ~33% efficiency, so if nuclear plants sold heat directly, it would reduce the cost per unit of energy by 1/3. This gives nuclear a strong economic advantage over electrified heat from renewable sources.
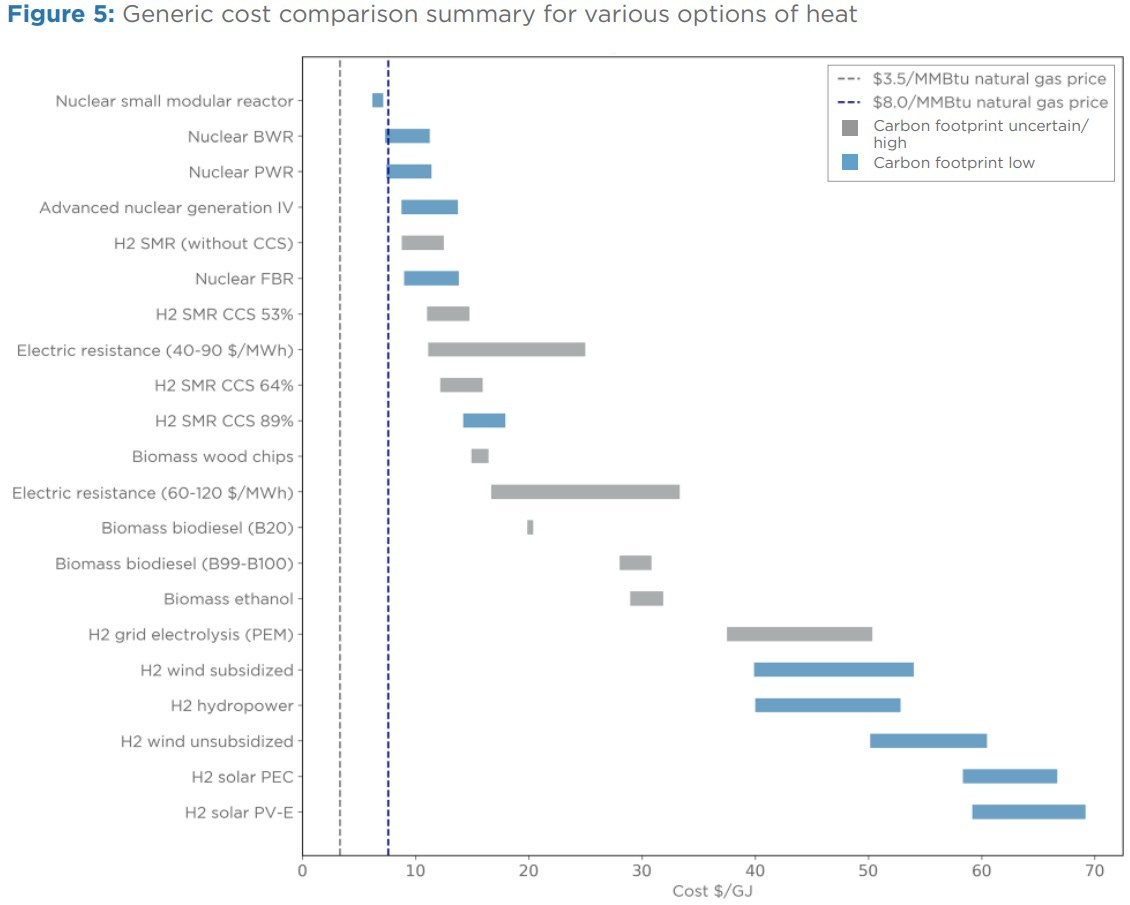
Historically, HTGRs have had the highest reactor outlet temperatures which will give them the most flexibility to meet the various demand cases. The HTTR in Japan is famous for a jaw-dropping 950°C core outlet temperature. In the ranges achievable for nuclear, there is almost 2X the demand for heat greater than 250°C than below which opens a much larger market size for high temperature reactors. The combination of the expected growing demand for zero-carbon high temperature heat and intrinsic cost advantage made a compelling case for HTGRs.
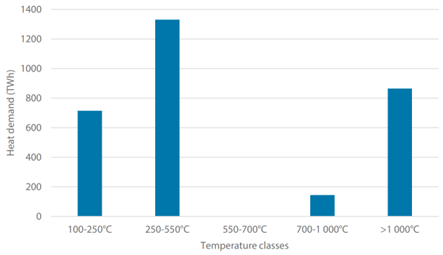
Slowing down MIGHTR activities…for now
From the beginning we said that we needed at least $1B in design and engineering before starting construction. Here is a screenshot from our first pitch deck in 2022. This was certainly sub-optimal from a fundraising perspective, but we wanted to partner with folks who had a realistic picture of what was required to deploy an advanced reactor.
In 2023, we did more in-depth planning, and we saw this was a lower bound. We are working on a future post that discusses the cost of designing multi-disciplinary machines, but in short, the NuScale plant and the AP1000 were both ~$1.5B design efforts, and they were incremental steps in pressurized water reactors. Advanced reactors are much more nascent, and lack active supply chains, and therefore we believe have higher development costs in today’s world.
Finally, the MIGHTR cost advantage was over other HTGRs, not LWRs. It is unclear if MIGHTR is cost-competitive with LWRs, or if any advanced reactor can be. There is a world where advanced reactors are equal or lower cost than LWRs, but it probably isn’t here yet, and until it is, we think capital should consolidate around deploying LWRs and developing the best advanced reactor concepts. In a future post we will discuss what needs to be true for advanced reactors to provide cheap, abundant power, and how to select the projects that would most likely affordably deploy advanced nuclear energy. Regarding MIGHTR, what we can say now is that we don’t expect to demonstrate any fueled version before the late 2030s.
Let’s work together
We transitioned Boston Atomics from a reactor development company to a nuclear R&D and consulting practice, with specialties in systems integration and techno-economic assessment for nuclear systems. In that vein, we have already partnered with national labs, universities, governments, reactor developers, and utilities. Reach out if you are interested.
In parallel, we are planning follow-up posts on topics like:
The cost to design complex machines
Kickstarting an advanced reactor industry
What’s needed for advanced reactors to be cost-competitive
The MIGHTR project
If you are in the advanced reactor ecosystem and have a topic that you have been itching to write about, please reach out: info@bostonatomics.com.